Cotton Fibre dyeing with Reactive Dyes in Neutral Media
“Extract from the paper published under
Department of Colour and Polymer Chemistry,The University of Leeds,
Leeds, LS2 9JT, UK“
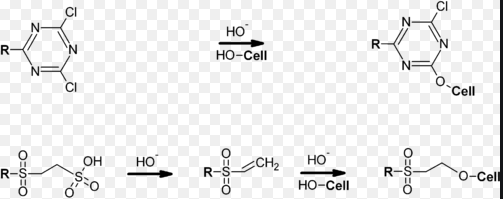
The most popular class of dyes for dyeing cotton are the reactive dyes; the reasons for this include brightness of shade, wide colour gamut, flexible application procedures and all-round good colour fastness properties of the resultant dyeings.
In the current long-liquor dyeing process it is common to apply the dyes in two definite steps:
- step 1- dye to equilibrium dye uptake (dye ‘exhaustion’) in the presence of a salt such as sodium chloride or sodium sulphate
- step 2 – achieve dye-fibre covalent bonding or ‘fixation’ by adding alkali. Dyeing under neutral conditions throughout would be advantageous since the dyer could avoid process interruptions to make alkali additions and the extent of reactive dye hydrolysis would be reduced due to the large reduction in the concentration of the competing nucleophile, the hydroxyl anion (HO-), in this neutral aqueous environment. A study of fundamental principles related to the dissociation of cellulose hydroxyl groups at high temperatures leads to a suitable methodology to achieve this objective. The dyebath is set with the required buffer at pH7.0-7.5 and raised to the appropriate temperature for covalent dye-fibre bonding (fixation) to occur; in this way, fixation values equivalent to or better than conventional processes are achievable along with dyeings of excellent levelness.
In 1983 Nippon Kayaku [1,2] marketed nicotinic acid quaternised triazine-based reactive dyes for dyeing under neutral conditions as the Kayacelon React range; these dyes were applied at pH7.5 at 100ºC for 1h, or at 130ºC in the case of deep shades Croft et.al. [3] demonstrated that other tertiary amines such as di-aza-bicyclo-octan (DABCO) could be used to produce neutral-fixing quaternised triazine dyes applicable to cotton at the boil. Lewis [4] indicated that the more cationic electrophilic site present in quaternised triazine dyes gives greater opportunity for close approach to the anionic nucleophile, Cell-O–, in the fibre, making a high degree of neutral fixation more likely compared with halo-s-triazine dyes applied under the same conditions.
As far as the authors are aware there has been no concerted effort to expand the principle of neutral fixation on unmodified cotton to all types of reactive dyes because of the physical chemistry of the cellulose/reactive dye system; in particular, for the cellulose-water system, there is a very low concentration of the reactive nucleophilic species, Cell-O–, unless the pH is raised, usually to within the region 10.5-12.0. The equation governing this dissociation may be written:
(Ka)
Cell-OH + H2O ⇄ Cell-O– + H3O+ …….(i)
where Ka is the dissociation constant of the conjugate acid Cell-OH; Ka is calculated according to the equilibrium concentrations of the various ionic species in equation (i):
Ka = [Cell-O–] [H3O+] ……………(ii)
[Cell-OH]
The higher the Ka value the greater the acid strength; clearly the acid strength of cellulose hydroxyl groups is low (according to Neale [5] Ka for Cell-OH is 1.84 x 10 –14 at 25○C). The ease of dissociation of an acid is often expressed as its pKa value which is the negative logarithm of its Ka value – in this case the smaller the value of pKa the stronger the acid.
At the point of half dissociation where [Cell-OH] = [Cell-O–], the pKa value is equal to the pH and thus pH titration is a very convenient method to obtain pKa values of various acids in aqueous media.
The extent of the dissociation of Cell-OH is clearly affected by the pH of the aqueous medium according to the following equation (iii):
Cell-OH + M+OH– ⇆ Cell-O-…..M+ + H2O …….(iii)
(where M+ is a suitable metal cation – usually Na+)
Equation (iii) is the reason why classical reactive dye application processes rely on alkali additions to increase the concentration of nucleophilic Cell-O– groups. Temperature increases can in principle achieve the same result since raising temperature from 25○C to 100○C can lower the pKa values of conjugate acids by up to two units [5] – i.e. increasing the concentration of Cell-O– one hundredfold. In this case ionization results in the formation of the species Cell-O-…..H3O+.
Dissociation of acids is also affected by ionic strength – thus an increase in salt concentration will increase the dissociation of the proton from the cotton cellulose hydroxyl residue.
All cotton reactive dyes on the market, with the exception of the Kayacelon React dyes or dyes having free vinylsulphone residues, have leaving groups which will behave as strong acids when a proton is picked up from the Cell-OH reaction zone; the nicotinic acid leaving group in the Kayacelon React dyes will not lower the local pH in this zone, since it can act as a buffer being an amino acid. The thesis was thus advanced that ensuring no drop in the pH of the internal fibre/aqueous medium should allow dye-fibre fixation under neutral conditions; dyeing and printing in the presence of moderate to high concentrations of buffer and at temperatures as high as 100○C was therefore studied.
Experimental
Materials
A bleached, all-cotton fabric (156g/m2) from Whalleys (Bradford, UK) was used throughout. Commercially available reactive dyes from various dye manufacturers were studied – they included the following:
Table 1. Dyes used in this study
Commercial name | C.I Generic name | Reactive groups |
Cibacron Red F-B | C.I Reactive Red 184 | Mono-amino-monofluorotriazine |
Cibacron Red LS-B | C.I Reactive Red 270 | Homo-bifluorotriazine |
Cibacron Blue LS-3R | C.I Reactive Blue 263 | Homo-bifluorotriazine |
Cibacron Brilliant Blue LS-G | C.I Reactive Blue 269 | Homo-bifluorotriazine |
Cibacron Yellow LS-R | C.I Reactive Yellow 208 | Homo-bifluorotriazine |
Remazol Black B | C.I Reactive Black 5 | Homo-bisulphatoethylsulphone |
Remazol Brilliant Blue R | C.I Reactive Blue 19 | Mono-sulphatoethylsulphone |
Remazol Red BS | C.I Reactive Red 22 | Mono-sulphatoethylsulphone |
Remazol Yellow 3RS | C.I Reactive Yellow 176 | Sulphatoethylsulphone |
Remazol Navy Blue GG | C.I Reactive Blue 203 | Sulphatoethylsulphone |
Remazol Brilliant Orange 3R | C.I Reactive Orange 16 | Monosulphatoethylsulphone |
Remazol Brilliant Green 6B | C.I Reactive Blue 38 | Sulphatoethylsulphone |
Remazol Brilliant Blue FB | C.I Reactive Blue 220 | Sulphatoethylsulphone |
Remazol Red RB | C.I Reactive Red 198 | Hetero-bifunctional |
Sumifix Supra Turq Blue BGF | C.I Reactive Blue 231 | Hetero-bifunctional |
Sumifix Supra Blue BRF | C.I Reactive Blue 221 | Hetero-bifunctional |
Sumifix Supra Yellow 3RF | C.I Reactive Yellow 145 | Hetero-bifunctional |
Sumifix Supra Navy Blue 3GF | C.I Reactive Blue 194 | Hetero-bifunctional |
Sumifix Supra Brilliant Red 3BF | C.I Reactive Red 195 | Hetero-bifunctional |
Procion Yellow HE-4R | C.I Reactive Yellow 84 | Bis-monochlorotriazine |
Procion Blue HE-RD | C.I Reactive Blue 160 | Bis-monochlorotriazine |
Procion Marine Blue HE-R | Bis-monochlorotriazine | |
Procion Red HE-3B | C.I Reactive Red 120 | Bis monochlorotriazine |
Procion Red MX-8B | C.I Reactive Red 11 | Dichlorotriazine |
Procion Blue MX-2G | C.I Reactive Blue 109 | Dichlorotriazine |
Procion Turquoise MX-G | C.I Reactive Blue 140 | Dichlorotriazine |
Procion Blue MX-4GD | C.I Reactive Blue 168 | Dichlorotriazine |
Procion Blue MX-G | C.I Reactive Blue 163 | Dichlorotriazine |
Procion Yellow MX-3R | C.I Reactive Orange 4 | Dichlorotriazine |
All other chemicals were of laboratory grade as supplied by Aldrich Chemicals (UK).
Application methods
Fabric samples were dyed at different temperatures in 100cm3 sealed stainless steel dyepots housed in a Mathis laboratory dyeing machine. The dyebaths were set with 2% (o.m.f) selected dyes and different concentrations of selected additives at various liquor-to-goods ratios. For conventional dyeing, the dyeing recipes are shown in table 2, with the time/temperature profiles for the Cibacron Red F-B, Cibacron LS and Remazol Black B applications being shown in Figures 1, 2, and 3, respectively.
Table 1. Dyeing recipes used in this study for conventional dyeing method
Dye | Sodium sulphate (g.dm-3) | Sodium carbonate (g.dm-3) |
Cibacron Red FB | 80 | 20 |
Cibacron LS | 25 | 12 |
Remazol Black B | 50 | 20 |
Cibacron Red F-B:
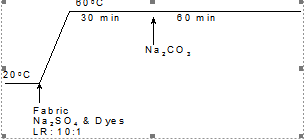
Figure 1. Time/temperature profile in the conventional dyeing of Cibacron Red FB
Cibacron LS:
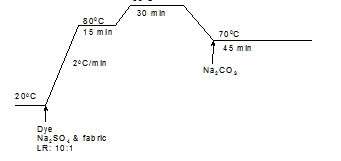
Figure 2. Time/temperature profile in the conventional dyeing process for Cibacron LS dyes
Remazol Black B:
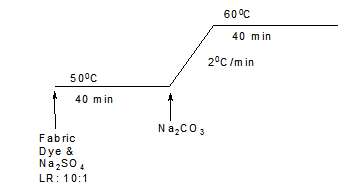
Figure 3. Time/temperature profile in the conventional dyeing process for Remazol Black B
In the case of the proposed neutral dyeing method, the time/temperature profile used for all the selected dyes in this study is shown in Figure 4.
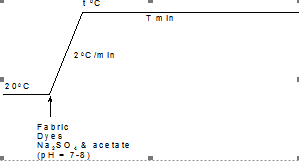
Figure 4. Time/temperature profile used when neutral dyeing the selected dyes
At the end of dyeing, the dyed fabrics were squeezed to return surplus dye liquor to the dyebath and then the dyebath, on cooling to ambient temperature, was analysed spectrophotometrically to obtain absorbance values at λmax. The dyed samples were rinsed under cold running water until no more colour desorbed, boiled with water for 15 min at 1000C, and washed in running cold water again until no further dye desorbed. The washed samples were dried at ambient temperature.
Determination of dye uptake ( exhaustion) and degree of dye-fibre covalent bonding (fixation)
The exhaustion achieved for a 2% dyeing on cotton fibres was determined using spectroscopic analysis of the dyebath before and after dyeing for different times. The concentration of dye in the dyebath was determined from the absorbance values at its lmax. The changes in absorbance versus concentration for each dye relies on the Lambert – Beer Law:
A = ε *C*l
Where: A – the absorbance of the dye at a particular wavelength,
C – the concentration of the dye, mol.dm-3
l – the pathlength of the cell used to measure the spectrum, cm.
ε – the molar extinction coefficient, dm3mol-1cm-1.
The percentage of dye exhaustion (%E) achieved for each dye was calculated from the following formula:
%E=
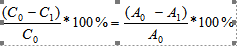
Where C0, C1 – the concentration of the dye before and after dyeing, respectively,
A0, A1 – the absorbance of the dyebath before and after dyeing, respectively.
The total fixation efficiency of each dyeing (%T) was calculated using the following formula:
%T=

Where C0, C1, C1’ – the concentration of the dye before and after dyeing and in the washed dyebath, respectively,
A0, A1, A1’ – the absorbance of the dyebath before and after dyeing, and of the wash-off liquors, respectively.
From the results of the dye exhaustion and the total efficiency of dye-fibre covalent bonding, the fixation of the dye absorbed (%F) was calculated for all dyeings by the given formula:
% F =

Results and discussion
The conventional dyeing methods were carried out to compare the results with those of the new dyeing method. The results displayed in Table 3 show the dyeing exhaustion, fixation ratio and total efficiency of dyes obtained from the conventional dyeing systems.
Table 2. Dyeing parameters produced by the conventional method
Dyes-2% o.m.f. | % E | % T | % F |
Cibacron Red F-B | 87.1 | 69.2 | 79.4 |
Cibacron Red LS-B | 79.9 | 68.1 | 85.3 |
Cibacron Blue LS-3R | 84.2 | 73.1 | 86.8 |
Cibacron Yellow LS-R | 93.3 | 87.7 | 94.0 |
Remazol Black B | 87.7 | 80.7 | 92.0 |
Effect of temperature
A series of neutral dyeings using Cibacron Red LS-B were carried out at 80, 90, 100, 110 and 120○C (1h, pH7, Na2SO4 80g dm-3, ± 40g dm-3 of various acetate salts).
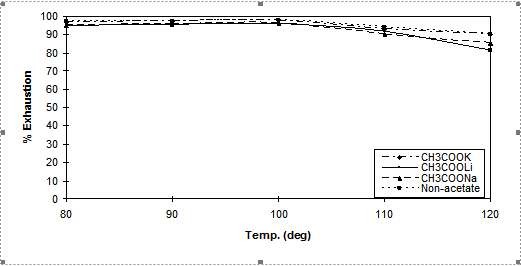
Figure 5. Dye exhaustion values versus temperature using 2% dye [Cibacron Red LS-B] applied using
80g.dm-3 of Na2SO4 and 40g.dm-3 of CH3COOM (M= Na, K, Li) and a LR 10:1
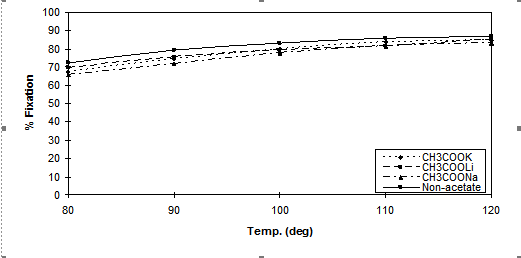
Figure 6. Fixation ratio versus temperature using 2% dye [Cibacron Red LS-B] applied using
80g.dm-3 of Na2SO4 and 40g.dm-3 of CH3COOM (M= Na, K, Li) and a LR 10:1
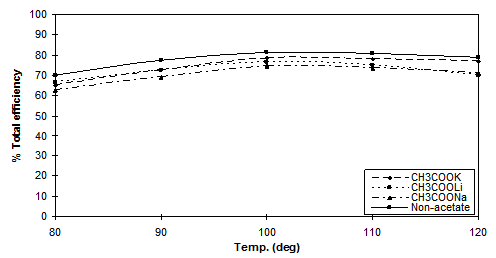
Figure 7. Total efficiency values versus temperature using 2% dye [Cibacron Red LS-B] applied
using 80g.dm-3 of Na2SO4 and 40g.dm-3 of CH3COOM (M= Na, K, Li) and a LR 10:1
The effects of temperature on dyeing properties are shown in Figures 5, 6 and 7. Temperature is one of the most important factors controlling the dyeing reaction. For all dyes, when the dyeing temperature was increased, the capillaries inside the fibre were more accessible and, as mentioned in the introduction, ionisation of Cell-OH to the nucleophilic Cell-O– is greatly increased, increasing the level of dye-fibre covalent bonding. Against this positive effect at high temperature the dye molecules have enhanced kinetic energy, causing rapid adsorption and desorption prior to covalent bonding resulting in lower substantivity.. However at very high dyebath temperatures (>110○C) dye hydrolysis reactions also occurred resulting in some loss of dye able to react with the fibre and a subsequent reduction in the value of total fixation efficiency obtained. Therefore, in neutral dyeing, the optimum temperature at which the total fixation efficiency is maximized depended upon the type of reactive group(s) present in the dyes.
Effect of electrolyte and acetate salt concentration
The role of electrolyte (either sodium sulphate and/or various metal acetates) was studied by measuring the total fixation efficiency values after dyeing Cibacron Red LS-B at 2% o.m.f. under neutral conditions for 1h at the boil; the results obtained are shown graphically in figure 8 (a, b and c)
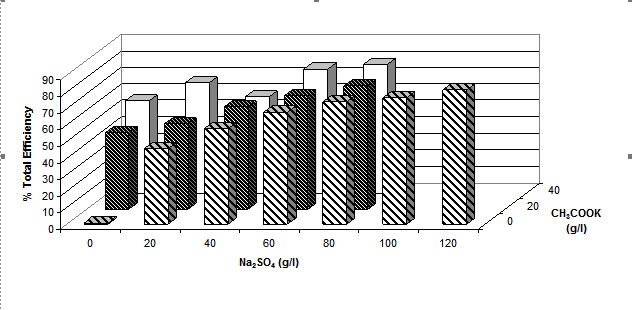
(a)
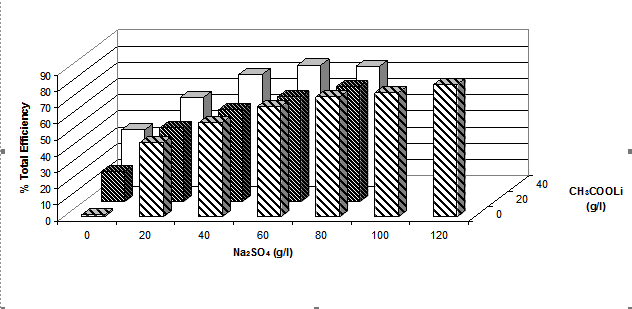
(b)
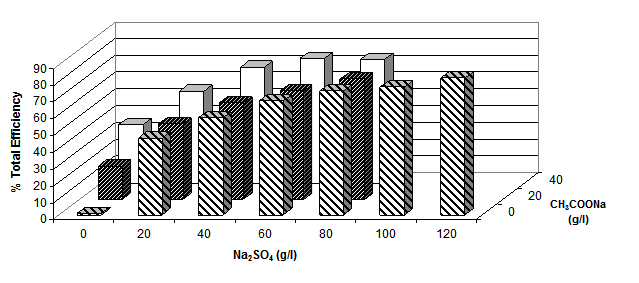
(c)
Figure 8. Total efficiency of 2% dye [Cibacron Red LS-B ] applied at 1000C using different sodium
sulphate concentrations and various concentrations of either (a) potassium acetate, (b)
lithium acetate or (c) sodium acetate at a LR 10:1
Figure 8 shows that the total fixation efficiency increased with an increase in the concentration of sodium sulphate and acetate salts. The water solubility of reactive dyes is high due to the ability of the sodium ion to dissociate from the sulphonate groups attached to the dye molecules. In their ionic state both the dye molecules and the cellulosate anions are negatively charged making it difficult for the dyes to adsorb onto the surface of the fibres and react with negatively charged cellulosate anions. By adding inert electrolyte, the electrostatic barrier can be suppressed and the adsorption of the dye molecules onto the fabric promoted. The sodium cations from the electrolyte adsorb onto the fibre surface and create a positive double layer, allowing the negative dye anions and the fibre with a positive layer to interact, explaining the increase in total fixation efficiency as the concentration of sodium sulphate increased.
It is also evident from the results that the greater the number of metal cations present in the acetate salts the higher the total dyeing efficiency; i.e. in 40g.dm-3 of a particular metal acetate salt, there is 10% of Li+, 28% of Na+ and 40% of K+. This means that, for the same amount of acetate salt used, potassium acetate appeared to have the greatest effect on the dyeing properties.
The acetate anion does not appear to play a role either as a catalyst for dye-fibre covalent fixation.
Effect of dyeing time
The effect of prolonging dyeing at the boil for Cibacron Red LS-B was studied and the results are shown in Figure 9.
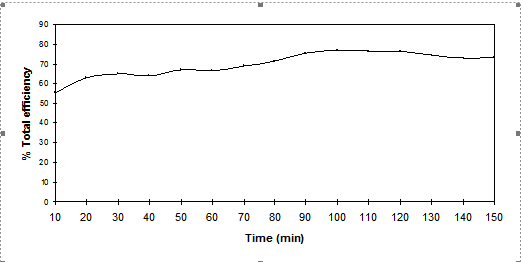
Figure 9. Total dyeing efficiency of Cibacron Red LS-B (C.I Reactive Red 270) at 1000C using 80g.dm-3 of
Na2SO4 and 40g.dm-3 of CH3COOK, dyeing for up to 150 min. at a LR 10:1
Covalent bond formation between the dye and the fibre can only occur when the dye has been adsorbed into the cellulose phase. The longer the dyeing time, the greater the amount of dye able to diffuse to the fibre surface, adsorb onto it, and react with cellulosate anions. However, adsorption is a reversible reaction; desorption tends to increase if the concentration of dye on the fibre surface is higher than that remaining in the dyebath; thus, the total fixation efficiency is reduced. In addition the hydrolysis of the dyes always competes with the desired fixation reaction. The longer the dyeing time, the more the dye is hydrolysed thus reducing fixation efficiency.
Effect of liquor-to-goods ratio
The effect of liquor ratio on dyeing parameters was determined using the dyes, other additives and physical conditions detailed in Table 4.
Table 3. Dyeing conditions
Dyes | Temp.(0C) | Time (min) | CH3COOK (g.dm-3) | CH3COOLi (g.dm-3) |
Cibacron Red F-B | 80 | 100 | 20 | |
Cibacron Red LS-B | 100 | 100 | 40 | |
Cibacron Blue LS-3R | 100 | 110 | 20 | |
Cibacron Yellow LS-R | 100 | 110 | 40 | |
Remazol Black B | 110 | 100 | 20 |
The results are shown graphically in Figure 10.
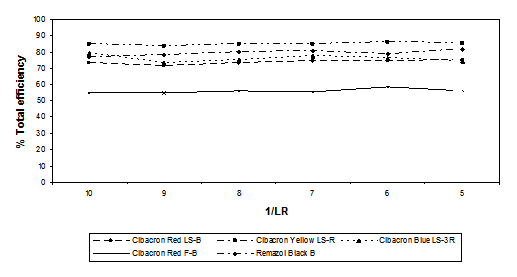
Figure 10. Total efficiency values [Cibacron Red LS-B, Cibacron Yellow LS-R, Cibacron Blue LS-3R Cibacron
Red F-B and Remazol Black B] versus liquor ratio
The new dyeing method also offers a chance to lower the liquor ratio; however, it must not be too low as to cause dye solubility problems and unlevelness. Higher liquor ratios decrease the possibility of contact between the dye molecules and the fibre surface. By changing the liquor ratio, the adsorption and desorption equilibrium is changed; conversely at lower liquor ratios more dye can be fixed onto the fibres.
Effect of reactive groups on dyeing properties
Table 5 shows the dye exhaustion values, fixation ratios and total fixation efficiency values for neutral dyeings using dyes with different reactive groups. The behaviour of the dyes varied depending on their subtantivity and reactivity. Cibacron LS dyes which contain the homo-bifluorotriazine reactive groups and Remazol dyes whose reactive groups are based on sulphatoethylsulphone either bifunctional (C.I Reactive Black 5) or monofunctional (C.I Reactive Blue 19) gave obviously good results in the presence of acetate salt in the dyebaths at the boil (1000C). However, Procion MX dyes, with the dichlorotriazinyl reactive group, possess such high reactivity that they readily hydrolyse at high temperature; the extent of fixation of these dyes is thus lower; in comparison the Procion HE class, based on lower reactivity bis-monochlorotriazine groups, have high subtantivity and thus give better overall efficiency but it is necessary, for the neutral dyeing system,to dye these at 140○C to achieve adequate dye-fibre covalent bonding. In the case of hetero-bifunctional dyes (Sumifix Supra) good fixation under neutral conditios was achieved when dyeing at the boil; similar good results were obtained for Remazol SES dyes applying them at the boil for 1h.
Table 4. Dyeing properties of dyes with various reactive groups under neutral application conditions
DYE | %E | %T | %F | Temp. (0C) |
Cibacron Red F-B | 93.7 | 54.6 | 58.3 | 80 |
Cibacron Red LS-B | 95.5 | 73.5 | 76.9 | 100 |
Cibacron Blue LS-3R | 96.5 | 79.2 | 82.1 | 100 |
Cibacron Brilliant Blue LS-G | 96.3 | 86.3 | 89.7 | 100 |
Cibacron Yellow LS-R | 98.9 | 84.9 | 85.8 | 100 |
Remazol Black B | 96.5 | 76.6 | 79.4 | 110 |
Remazol Brilliant Blue R | 86.8 | 62.3 | 71.8 | 100 |
Remazol Red BS | 76.9 | 28.4 | 37.0 | 100 |
Remazol Yellow 3RS | 91.7 | 76.0 | 82.9 | 100 |
Remazol Navy Blue GG | 97.5 | 88.2 | 90.5 | 100 |
Remazol Brilliant Orange 3R | 73.3 | 47.7 | 65.1 | 100 |
Remazol Brilliant Green 6B | 93.3 | 77.5 | 83.1 | 100 |
Remazol Brilliant Blue FB | 94.4 | 84.3 | 89.4 | 100 |
Remazol Red RB | 74.0 | 53.3 | 72.0 | 100 |
Sumifix Supra Turq Blue BGF | 95.0 | 48.1 | 50.7 | 100 |
Sumifix Supra Blue BRF | 95.9 | 66.6 | 69.4 | 100 |
Sumifix Supra Yellow 3RF | 89.0 | 58.0 | 65.2 | 100 |
Sumifix Supra Navy Blue 3GF | 92.3 | 67.6 | 73.2 | 100 |
Sumifix Supra Brilliant Red 3BF | 76.4 | 54.6 | 71.7 | 100 |
Procion Yellow HE-4R | 77.6 | 63.2 | 81.4 | 140 |
Procion Blue HE-RD | 90.9 | 78.7 | 86.5 | 140 |
Procion Marine HE-R | 79.6 | 60.9 | 76.5 | 140 |
Procion Red HE-3B | 82.4 | 62.3 | 75.6 | 140 |
Procion Red MX-8B | 90.1 | 33.13 | 36.8 | 60 |
Procion Blue MX-2G | 77.2 | 29.2 | 37.9 | 60 |
Procion Turquoise MX-G | 96.0 | 55.4 | 57.7 | 100 |
Procion Blue MX-4GD | 96.2 | 53.5 | 55.6 | 60 |
Procion Blue MX-G | 85.0 | 62.1 | 73.1 | 100 |
Procion Yellow MX-3R | 74.5 | 58.5 | 78.6 | 140 |
Conclusions
A study of neutral fixation (pH7) of a variety of reactive dyes on cotton has demonstrated that promising overall total fixation efficiencies can be obtained by increasing electrolyte concentration, by working at the lowest possible liquor ratios and by dyeing at 100○C for fluoro-triazine and vinylsulphone derivatives and at 140○C for the less reactive monochloro-triazine derivatives. Because of the neutral dyeing conditions, the risk of hydrolysis of dyes can be minimized. Even in this new dyeing process, the temperature, and the concentration of electrolyte used are higher than those in the conventional dyeing method. However because of the low liquor ratio employed, less energy is used to heat the dyebath solution and a lower amount of chemicals is used. The smaller volume of water used for dyeing, and reduction in waste effluents potentially reduces the cost of waste water treatment after dyeing. The neutral exhaust application of reactive dyes to cellulosic fibres gave dyeings with excellent reproducibility, levelness, and penetration.
Moreover, since all the chemicals such as dyes, electrolyte, and acetate can be put into the dyeing solution at the same time, it is possible to simplify the dyeing process and save operation costs.
The work carried out for this study has been on a bench scale, but the results obtained show promise for commercial scale application.
References
- Morimura, N., and Ojima, M., Amer. Dyestuff Rep., 74 (1985).
2. Miyamoto, N., and Parkham, R., AATCC Int. Conf. & Exhib., (1986) 153.
3. Croft, S.N., Lewis, D. M., Orita, R., and Sugimoto, T., J Soc. Dyers & Col.,
108 (1992) 195.
- Lewis, D. M., Adv. in Col. Sci. & Techn., 4 (2001) 41.
- Neale, S. M., J Text Inst, 21 (1930) 225-230.
- Altenhofen, U., PhD Thesis, Deutsches Wollforschungs Institut (Aachen) 1977.